Solar-Powered Microgrids Transform Urban Mobility in Europe
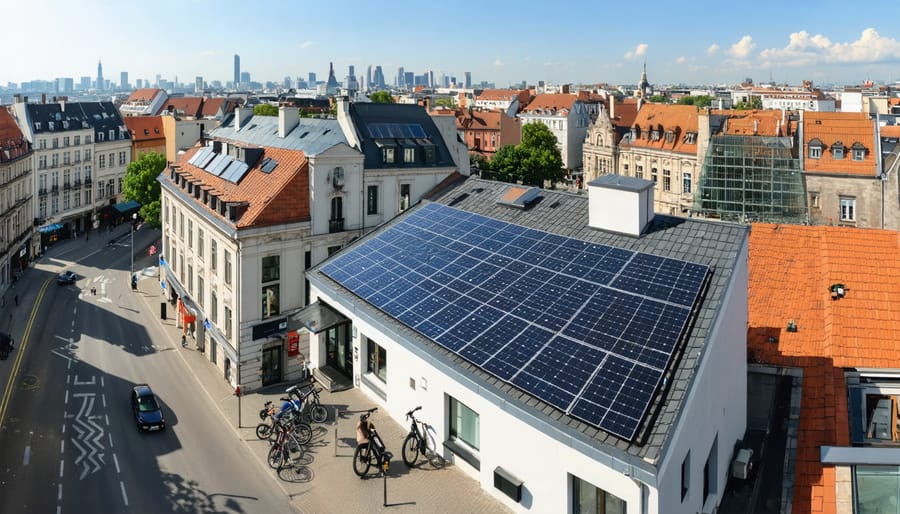
Solar-powered microgrids are emerging as one of Europe’s most game-changing energy systems, revolutionizing how communities and businesses achieve energy independence. These localized power networks combine advanced solar technology, smart storage solutions, and sophisticated control systems to create resilient, sustainable energy ecosystems. By integrating renewable energy generation with intelligent distribution networks, microgrids offer unprecedented control over power consumption while significantly reducing carbon emissions and operating costs. For European organizations pursuing energy autonomy and sustainability goals, solar microgrids represent a strategic investment that delivers immediate benefits while future-proofing energy infrastructure against growing climate challenges and rising utility costs. From remote villages to urban industrial complexes, these systems are reshaping the energy landscape, providing reliable, clean power while supporting the EU’s ambitious climate targets and energy security objectives.
How Solar Microgrids Power Modern Mobility
Core Components of Solar Microgrids
Solar microgrids rely on three essential components that work together seamlessly to deliver reliable and sustainable power. At the heart of these systems are high-efficiency solar panels, which convert sunlight into electricity through photovoltaic technology. Modern solar arrays are designed to maximise energy capture even in varying European weather conditions, featuring advanced materials and smart tracking systems that optimise their positioning throughout the day.
Energy storage systems form the crucial second component, typically comprising advanced lithium-ion batteries or other innovative storage solutions. These systems store excess energy generated during peak sunlight hours for use during nights or cloudy periods, ensuring continuous power availability. The storage capacity is carefully calculated to match local energy consumption patterns and backup power requirements.
The third vital component is the smart distribution system, which includes sophisticated power electronics and control mechanisms. This intelligent network manages power flow, automatically switching between solar, stored, and grid power as needed. It incorporates monitoring equipment and software that optimise energy distribution, prevent outages, and enable remote system management. Modern distribution systems also feature built-in safety protocols and can seamlessly integrate with existing electrical infrastructure, making them ideal for European commercial and residential applications.
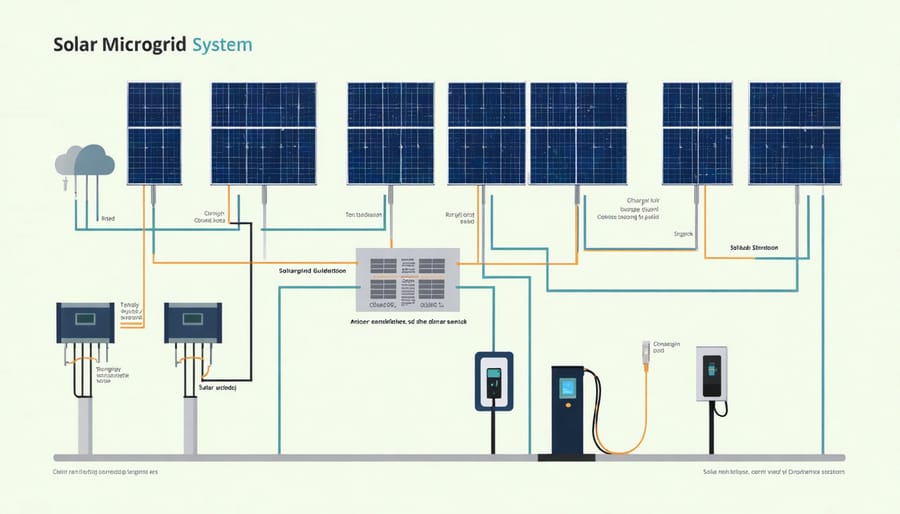
Integration with Micro-Mobility Hubs
The integration of solar microgrids with micro-mobility solutions represents a significant advancement in sustainable urban transportation. These systems seamlessly connect with e-bike stations, scooter-sharing points, and other mobility hubs through sophisticated solar charging infrastructure, creating an efficient ecosystem for clean transportation.
Modern microgrid installations feature smart power management systems that automatically distribute energy based on demand patterns. During peak sunlight hours, excess solar power charges mobility devices, while battery storage systems ensure consistent power availability during cloudy periods or nighttime. This integration supports the growing network of shared mobility services across European cities.
The system’s intelligent load management ensures optimal power distribution between different charging points, prioritising fast-charging capabilities during high-demand periods. Real-time monitoring allows operators to track energy consumption, charging status, and system performance through user-friendly interfaces. This data-driven approach helps optimize energy usage and maintain reliable service for micro-mobility users while maximizing the benefits of renewable energy integration.
By connecting these mobility hubs to solar microgrids, communities create self-sustaining transportation networks that reduce reliance on traditional power grids while promoting eco-friendly urban mobility solutions.
Real-World Implementation Success Stories
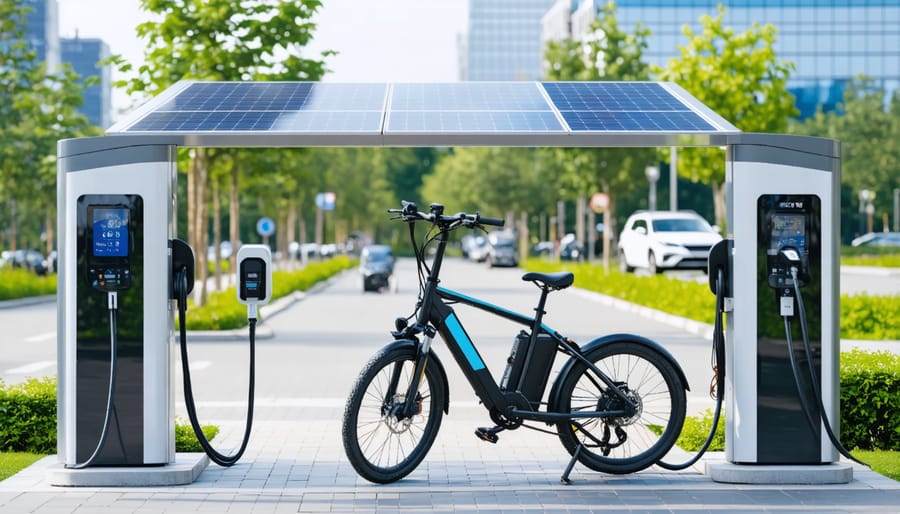
Urban Integration Examples
Several European cities showcase successful integration of solar-powered microgrids into their urban infrastructure. Amsterdam’s Solar City project incorporates over 50 distributed microgrids across residential neighborhoods, powering both homes and sustainable urban transit solutions. The system has reduced grid dependency by 40% while enhancing community resilience during power disruptions.
In Barcelona, the Industrial District Microgrid powers 200 businesses through a combination of rooftop solar arrays and smart energy management systems. This installation demonstrates how urban industrial zones can achieve greater energy independence while reducing carbon emissions by approximately 3,000 tonnes annually.
Helsinki’s Smart Solar District represents another innovative implementation, where interconnected microgrids serve multiple apartment complexes, offices, and public facilities. The project incorporates advanced energy storage solutions and intelligent load management, achieving 60% solar energy utilization even during winter months.
Milan’s Sustainable Quarter project exemplifies how historic European cities can seamlessly integrate solar microgrids while preserving architectural heritage. The system powers 1,500 residential units and includes public charging stations for electric vehicles, successfully balancing modern energy needs with cultural preservation.
Performance Metrics and Benefits
Solar powered microgrids demonstrate impressive performance metrics across multiple sustainability indicators. Studies show that well-designed systems can achieve energy self-sufficiency rates of up to 85-95% during peak solar hours, significantly reducing grid dependency. In terms of carbon footprint reduction, a typical commercial microgrid installation can offset approximately 50-100 tonnes of CO2 emissions annually, depending on system size and location.
Cost-efficiency data from European installations reveals average energy cost savings of 30-40% compared to traditional grid power, with some optimised systems reaching up to 50% reduction in energy expenses. The return on investment (ROI) typically ranges from 5-8 years, influenced by local solar conditions and energy prices.
Performance reliability is another crucial metric, with modern solar microgrids maintaining 99.9% uptime through intelligent energy management systems. Energy storage solutions integrated within these systems can provide 24-48 hours of backup power, ensuring continuous operation during adverse weather conditions.
Environmental benefits extend beyond emissions reduction. These systems reduce transmission losses by up to 7% compared to centralised power distribution, while their modular nature allows for scalable expansion with minimal environmental disruption. Water conservation is another advantage, as solar microgrids consume virtually no water during operation, unlike traditional power generation methods.
Smart Energy Management Systems
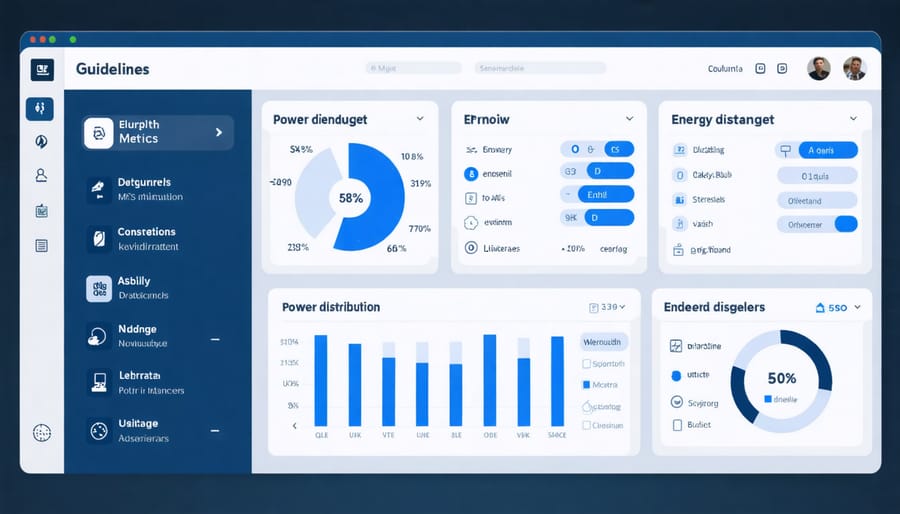
Demand Response Systems
Demand Response Systems form the intelligent backbone of solar powered microgrids, enabling optimal energy distribution and consumption patterns. These smart systems continuously monitor energy demand, production, and storage levels, automatically adjusting power flow to maintain grid stability and efficiency.
During peak usage periods, demand response mechanisms automatically implement pre-programmed strategies to reduce strain on the system. This might include temporarily reducing non-essential power consumption, activating stored energy reserves, or redistributing available power to priority areas. The system can also incentivise users to shift their energy consumption to off-peak hours through dynamic pricing models and automated controls.
Advanced algorithms analyse historical usage patterns, weather forecasts, and real-time data to predict demand spikes and optimize energy distribution accordingly. This predictive capability allows the microgrid to prepare for high-demand periods by increasing energy storage or adjusting production schedules.
For European businesses and households, these systems typically integrate with smart meters and building management systems, offering detailed consumption analytics and automated energy-saving recommendations, while ensuring compliance with local grid codes and regulations.
Grid Stability and Reliability
Grid stability and reliability are crucial aspects of solar-powered microgrids, especially when supporting mobility services. Advanced power management systems incorporate smart inverters and sophisticated control algorithms to maintain voltage and frequency within optimal ranges. These systems continuously monitor power quality and automatically adjust to fluctuations in both supply and demand.
Energy storage solutions, particularly lithium-ion batteries and emerging storage technologies, play a vital role in maintaining grid stability. They store excess solar energy during peak production hours and release it when needed, ensuring a consistent power supply even during cloudy periods or nighttime.
Load balancing mechanisms distribute power efficiently across the microgrid, preventing system overload and maintaining service quality. Real-time monitoring systems detect potential issues before they affect operations, while automated switching systems can isolate problems and reroute power as needed.
For enhanced reliability, many European microgrids implement redundancy measures and backup systems. These might include secondary power sources or interconnections with neighbouring microgrids, ensuring continuous operation of critical mobility services even during maintenance or unexpected events.
Future Development and Scalability
The future of solar-powered microgrids holds tremendous potential for expansion and technological advancement across Europe. As battery storage technology continues to evolve, we’re seeing significant improvements in energy density and cost-effectiveness. Experts predict that by 2025, battery costs will decrease by up to 40%, making microgrid solutions increasingly accessible to communities and businesses.
Artificial Intelligence and machine learning are revolutionising microgrid management systems, enabling more precise energy distribution and predictive maintenance. These smart systems can anticipate energy demands, optimise storage, and automatically adjust to weather patterns, significantly improving overall efficiency.
Innovation in solar panel technology is equally promising, with new-generation panels achieving higher conversion rates while requiring less space. Bifacial panels, which can capture sunlight from both sides, are becoming more prevalent, offering up to 30% more energy generation in optimal conditions.
Scalability is being enhanced through modular design approaches, allowing microgrids to grow alongside community needs. This flexibility enables gradual expansion without significant disruption to existing systems, making long-term planning more practical and cost-effective.
European initiatives are focusing on developing standardised protocols for microgrid integration, which will facilitate easier connection to main grids and between different microgrids. This interconnectivity will create more resilient energy networks and support the EU’s renewable energy targets.
Looking ahead, emerging technologies like solid-state batteries and advanced power electronics will further enhance microgrid capabilities. Combined with ongoing developments in energy management software and increasing government support, solar-powered microgrids are positioned to play a crucial role in Europe’s sustainable energy future.
Solar-powered microgrids are revolutionizing micro-mobility across Europe, offering sustainable solutions that align perfectly with the continent’s ambitious climate goals. The integration of these systems has demonstrated remarkable success in reducing carbon emissions while providing reliable power for electric vehicle charging stations, e-bike sharing networks, and other micro-mobility infrastructure.
As we look toward the future, the potential for growth is substantial. Cities across Europe are increasingly adopting these solutions, with projections indicating a significant expansion in the next decade. The combination of advancing solar technology, improved energy storage capabilities, and declining installation costs makes these systems increasingly attractive for municipalities and businesses alike.
The impact extends beyond environmental benefits, creating new opportunities for local energy independence and economic growth. Many European communities are already experiencing reduced operating costs and enhanced grid resilience through these implementations. With continued technological innovation and supportive policy frameworks, solar-powered microgrids are set to play a crucial role in shaping sustainable urban mobility solutions.
As we move forward, these systems will become increasingly sophisticated, incorporating smart grid technologies and advanced energy management systems, further optimizing their performance and reliability for micro-mobility applications across Europe.
Leave a Reply